The Role of Cell Membrane Lipids in General Anesthesia
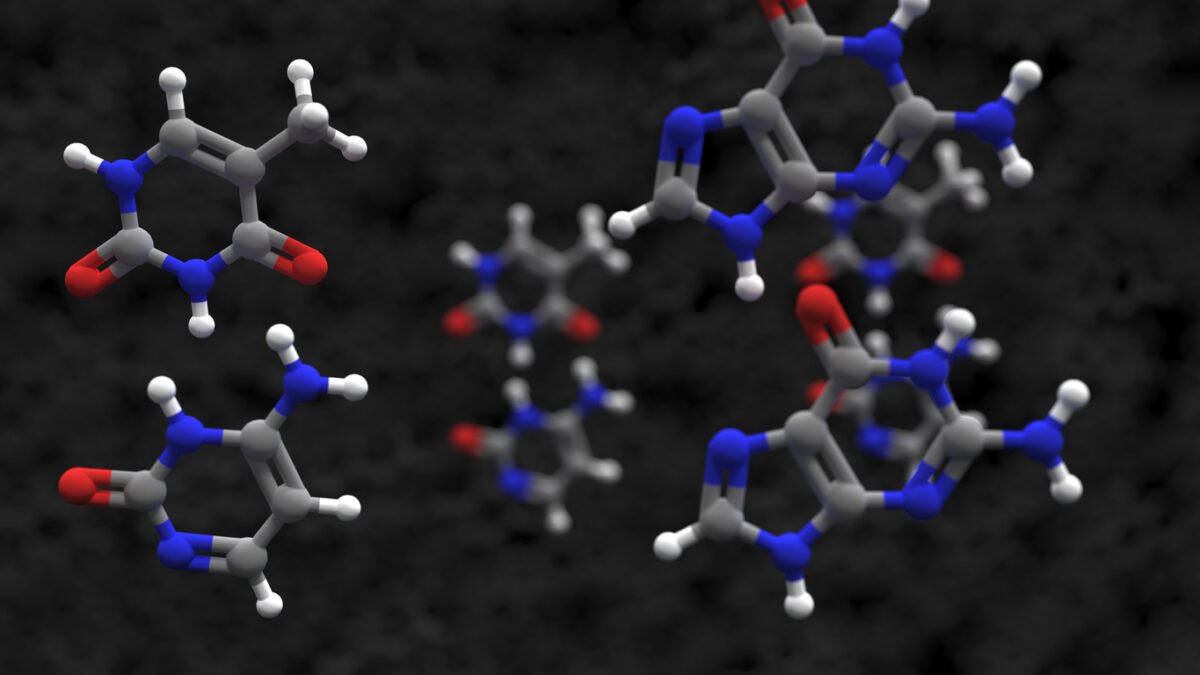
Though general anesthesia was first developed in 1846, scientists and doctors still lack a robust understanding of anesthesia’s molecular functionality [1]. Because of the Meyer-Overton correlation, which identifies lipophilicity as the most important indicator of an anesthetic’s potency, scientists agree that there must be a connection between anesthesia and cell membrane lipids [1, 2]. As a result, many have hypothesized that lipids are a crucial intermediary between anesthesia inhalation and the activation of TWIK-related potassium channels, or TREK-1, which diminish pain [3].
Scientists are largely in agreement about the general steps leading from anesthetic inhalation to TREK-1 activation. PLD2, or phospholipase D2, is the essential enzyme that prompts the production of phosphatidic acid (PA), which in turn activates TREK-1 [3]. In studies where the PLD2 of mice are replaced by catalytically dead PLD2 mutants, activation of TREK-1 channels becomes impossible and anesthesia is completely ineffective [4]. These findings demonstrate that anesthesia induces unconsciousness via PLD2 [4]. The remaining question is to elucidate the full mechanism of general anesthesia.
Inhaled anesthetics, such as isoflurane and chloroform, are known to disrupt lipid rafts [1]. One such lipid raft is GM1 [1]. Lipid rafts are membrane regions with high, orderly concentrations of certain lipids [4]. When inhaled anesthetics enter the body, their diverse set of chemical compounds augment the quantity and size of lipids in GM1 domains by increasing concentrations of cholesterol [4, 5]. Despite this noticeable effect, studies comparing the effects of mechanical force and anesthetics on GM1 domains proved that this could not be the method through which anesthesia transports PLD2 away from GM1 domains [4]. Mechanical force and anesthesia both have similar effects on TREK-1, suggesting that they affect the same intermediaries [4]. However, because mechanical force leads to a reduction in the lipid quantities in GM1 domains–in short, producing an opposite effect as anesthesia–this hypothesis is considered incomplete [4].
A new theory refines the previous hypothesis by adding an additional step. First, anesthesia disrupts GM1 domain structure by expanding lipid rafts [4]. Then, these severe changes in lipid structure similarly perturb palmitate-mediated localization of PLD2 to GM1 lipids [4]. The result is PLD2 translocation: PLD2 is transported away from GM1 domains and binds to PIP2 lipids, which cues substrate presentation and, ultimately, production of PA [4]. Comparative studies of mechanical force and anesthesia support this conclusion because, whether GM1 domains are expanded or reduced, palmitate-mediated localization is still affected [4]. Additionally, this two-step understanding clarifies how PLD2 can result in either the activation or inhibition of TREK-1, which have been observed in different contexts [4].
Ultimately, this refined understanding of how anesthesia affects the lipids in cell membranes is a significant step forward in understanding the complexities of general anesthesia [3]. However, the connection between mechanical force and anesthesia should be analyzed further to determine whether their differing effects on GM1 domains suggest the existence of another, yet to be discovered intermediary [1]. Regardless, the current membrane-mediated mechanism is important to consider in investigating further protein-anesthesia interactions.
References
[1] M. A. Pavel et al., “Studies on the mechanism of general anesthesia,” Proceedings of the National Academy of Sciences of the United States of America, vol. 117, no. 24, p. 13757-13766, June 2020. [Online]. Available: https://doi.org/10.1073/pnas.2004259117. [Accessed October 9, 2020].
[2] H. Tsuchiya and M. Mizogami, “Interaction of Local Anesthetics with Biomembranes Consisting of Phospholipids and Cholesterol: Mechanistic and Clinical Implications for Anesthetic and Cardiotoxic Effects, Anesthesiology Research and Practice, vol. 2013, no. 4, September 2013. [Online]. Available: https://doi.org/10.1155/2013/297141. [Accessed October 9, 2020].
[3] M. A. Pavel, H. W. Chung, N. E. Petersen, S. B. Hansen, “Polymodal Mechanism for TWIK-Related K+ Channel Inhibition by Local Anesthetic,” Anesthesia & Analgesia, vol. 129, no. 4, p. 973-982, October 2019. [Online]. Available: https://doi.org/10.1213/ANE.0000000000004216. [Accessed October 9, 2020].
[4] E. N. Petersen, M. A. Pavel, H. Wang, and S. B. Hansen, “Disruption of palmitate-mediated localization; a shared pathway of force and anesthetic activation of TREK-1 channels,” Biochimica et Biophysica Acta (BBA) – Biomembranes, vol. 1862, no. 1, January 2020. [Online]. Available: https://doi.org/10.1016/j.bbamem.2019.183091. [Accessed October 9, 2020].
[5] G. Hantal, B. Fábián, M. Sega, B. Jójárt, P. Jedlovsky, “Effect of general anesthetics on the properties of lipid membranes of various compositions,” Biochimica et Biophysica Acta (BBA) – Biomembranes, vol. 1861, no. 3, p. 594-609, March 2019. [Online]. Available: https://doi.org/10.1016/j.bbamem.2018.12.008. [Accessed October 9, 2020].