Pharmacology of Sevoflurane
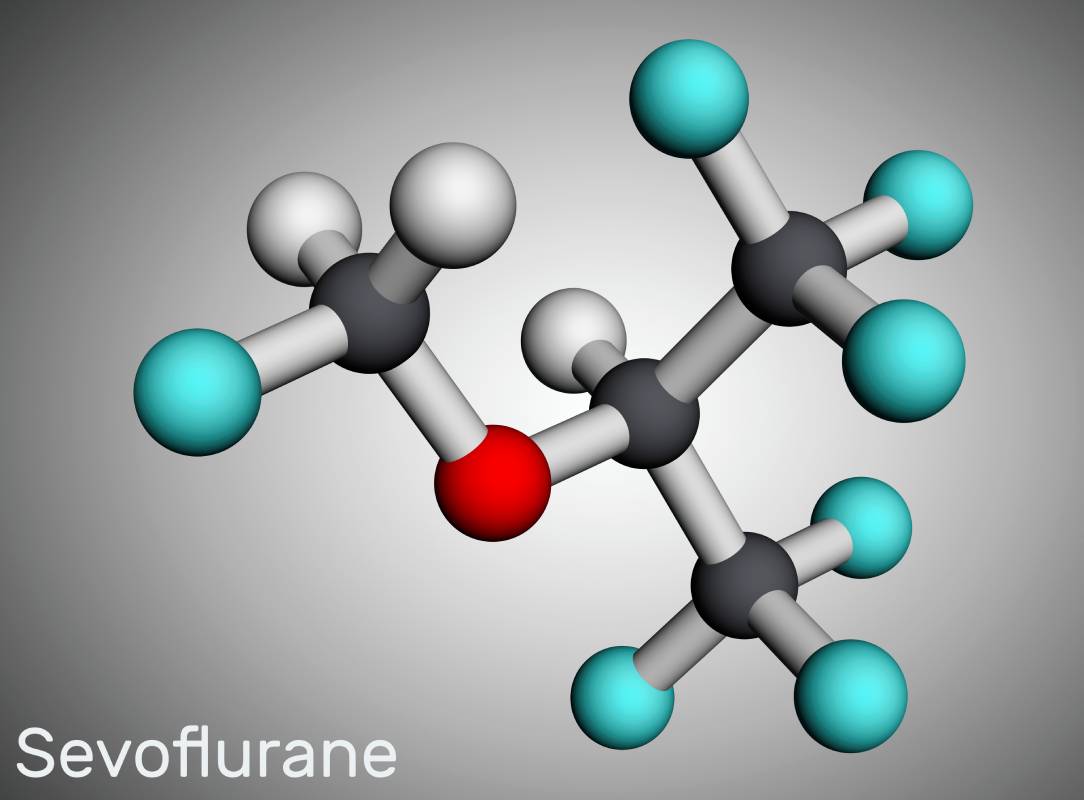
Halogenated inhalational anesthetics are among the most widely employed anesthetic agents in both adults and pediatric patients undergoing surgical procedures. Sevoflurane is an inhalational anesthetic with several advantageous properties, facilitating smooth induction and making the drug especially amenable for rapid induction of anesthesia in patients.1 The unique pharmacology of sevoflurane drives its widespread use.
One of the main neural targets of sevoflurane is the glutamate system, whose receptors are involved in excitatory neurotransmission. Sevoflurane decreases the activity of these receptors, which results in anesthetic effects such as muscle relaxation and attenuation of autonomic responses to surgical stimuli.2 Interestingly, a study analyzing the micro-circuitry of sevoflurane pharmacology found the anesthetic significantly decreased NMDA glutamate receptor activity but had no effect on AMPA glutamate receptors.3 To assess sevoflurane’s recovery period, the researchers examined glutaminergic currents during anesthetic perfusion and the subsequent wash-out. NMDA peak currents started to decrease around 50 seconds after the beginning of the perfusion and reached steady state level in about 140 seconds. In response to the anesthetic wash out, excitatory currents started to increase again after about 100 seconds.3
Sevoflurane’s pharmacology also increases the activity of the gamma-aminobutyric acid type A (GABA-A) receptor, the primary inhibitory neurotransmitter receptor in the central nervous system. This causes hyperpolarization of postsynaptic neurons, in turn inhibiting neuronal firing. The resulting state leads to the characteristic effects of anesthesia, including hypnosis, amnesia, and analgesia. Voltage clamping studies demonstrated spontaneous inhibitory post-synaptic currents (sIPSCs) could be found in cells that had received sevoflurane anesthesia. Recordings also showed significant potentiation of GABAergic granule cells after sevoflurane.3 Analyses of GABAergic current properties during anesthetic perfusion and wash-out showed a more rapid change in the inhibitory currents compared to the excitatory ones. GABAergic currents started to increase around 30 seconds after the beginning of the perfusion and reached steady state in less than 100 seconds. In response to the anesthetic wash out, GABAergic currents began decreasing after 50 seconds.3 These results indicate while sevoflurane has a significant effect on both excitatory and inhibitory neurotransmission, it has a more rapid effect on inhibitory neurotransmission.
Sevoflurane exerts effects on other biological systems as well. In adults, sevoflurane depresses cardiovascular function by decreasing myocardial contractility. This effect may be attenuated with concurrent administration of nitrous oxide or with prolonged exposure to sevoflurane.1 Sevoflurane also has a dose-dependent effect on the respiratory system, inhibiting hypoxic pulmonary vasoconstriction and tracheal smooth muscle contraction. Sevoflurane may also suppress natural inflammatory responses during one-lung ventilation procedures.4
Like other volatile anesthetics, sevoflurane can potentially cause hepatotoxicity through the oxidation of sevoflurane in the liver by the enzyme CYP2E. The resulting metabolite, called fluoroacetic acid, may modify liver microsomal proteins, creating an unnecessary immune response and resulting in hepatic dysfunction. In volunteer studies, sevoflurane has also been shown to increase markers of nephrotoxicity, such as urinary albumin, urinary glucose, and alpha-glutathione-S-transferase (GST).5
Through strong communication, collaboration, and coordination, healthcare providers around the world can reduce risks, manage complications, and deliver high-quality care with anesthesia that is well tailored to every patient’s specific needs. Considering the research on sevoflurane’s pharmacology and effect on neighboring biological systems, emphasizing patient safety is crucial to achieving successful outcomes and optimizing the well-being of patients in clinical settings.
References
- Patel, Sanjay S., and Karen L. Goa. “Sevoflurane.” Drugs, vol. 51, no. 4, Apr. 1996, pp. 658–700. https://doi.org/10.2165/00003495-199651040-00009
- Karunarathna, I., Kusumarathna, K., Gunarathna, I., & Kurukulasooriya, P. Sevoflurane: Pharmacology, Administration, and Safety Considerations in Anesthesia. 2024.
- Mapelli, Jonathan, et al. “The Effects of the General Anesthetic Sevoflurane on Neurotransmission: An Experimental and Computational Study.” Scientific Reports, vol. 11, Feb. 2021, p. 4335. https://doi.org/10.1038/s41598-021-83714-y
- Sugasawa, Yusuke, et al. “Effects of Sevoflurane and Propofol on Pulmonary Inflammatory Responses during Lung Resection.” Journal of Anesthesia, vol. 26, no. 1, Feb. 2012, pp. 62–69. https://doi.org/10.1007/s00540-011-1244-y
- Eger, Edmond I., et al. “Nephrotoxicity of Sevoflurane Versus Desflurane Anesthesia in Volunteers:” Anesthesia & Analgesia, vol. 84, no. 1, Jan. 1997, pp. 160–68. https://doi.org/10.1097/00000539-199701000-00029